Library
Ammonia Disposal – Short-answer questions
- November 16, 2024
- Posted by: Namrata Chhabra
- Category: Amino acid metabolism Library Metabolism of Amino acids Short-Answer questions USMLE Content
Question 1: What is the primary function of the urea cycle in humans?
Answer: The urea cycle converts ammonia, a highly toxic byproduct of amino acid metabolism, into urea, a less toxic compound that can be safely excreted in urine. This process is vital for maintaining nitrogen balance in the body.
Question 2: Which cellular compartment houses the enzymes responsible for the urea cycle?
Answer: The urea cycle takes place across two cellular compartments: the mitochondria and the cytosol of liver cells. Some reactions occur within the mitochondrial matrix, while others take place in the cytosol.
Question 3: What is the role of N-acetylglutamate in regulating the urea cycle?
Answer: N-acetylglutamate acts as an allosteric activator of carbamoyl phosphate synthetase I, the enzyme responsible for the first committed step of the urea cycle. This activation increases the rate of urea synthesis when necessary, such as during periods of increased protein breakdown.
Question 4: What are the common clinical features associated with urea cycle disorders?
Answer: Urea cycle disorders lead to a buildup of ammonia in the bloodstream, resulting in hyperammonemia. This can cause a variety of symptoms, including vomiting, lethargy, seizures, coma, and mental retardation.
Question 5: Apart from dietary restrictions, what other treatment strategies can be employed to manage urea cycle disorders?
Answer: In addition to limiting protein intake, treatment may involve administering compounds that scavenge nitrogen, such as sodium benzoate or sodium phenylbutyrate. These compounds help to remove excess nitrogen from the body through alternative pathways, reducing the burden on the impaired urea cycle. Gene therapy as a potential treatment strategy is under investigation, but it is not currently an effective option for human subjects.
Question 6: What are the primary sources of ammonia in the human body?
Answer: Ammonia in the body arises primarily from the α-amino nitrogen of amino acids. Sources of ammonia in the body include the breakdown of amino acids in tissues and the ammonia produced by enteric bacteria that is absorbed into portal venous blood.
Question 7: Why is ammonia considered toxic, particularly to the central nervous system?
Answer: Ammonia is considered toxic, particularly to the central nervous system (CNS), due to the following key reasons:
- Disruption of Glutamate-Glutamine Cycle
- Ammonia readily crosses the blood-brain barrier and reacts with glutamate to form glutamine via the enzyme glutamine synthetase.
- This reaction reduces glutamate levels in the brain, which is critical for:
- Excitatory neurotransmission (glutamate is a major excitatory neurotransmitter).
- GABA synthesis (as glutamate is a precursor for GABA, an inhibitory neurotransmitter).
- A decrease in glutamate and GABA disrupts the balance of excitatory and inhibitory signals, impairing neural function.
- Altered Energy Metabolism
- Ammonia inhibits key enzymes in the TCA cycle (e.g., α-ketoglutarate dehydrogenase) by depleting α-ketoglutarate, a vital TCA intermediate.
- This reduces ATP production in neurons, impairing their ability to maintain normal ion gradients and synaptic activity.
Clinical Manifestations
- Symptoms of ammonia toxicity often include confusion, irritability, lethargy, seizures, and in severe cases, coma or death. These are hallmark features of hepatic encephalopathy or urea cycle disorders.
Protective Mechanisms
- The body has mechanisms to manage ammonia, such as urea synthesis in the liver and glutamine synthesis in the brain. When these mechanisms are overwhelmed, ammonia toxicity ensues.
Question 8: How does liver dysfunction contribute to the development of ammonia intoxication?
Answer: Liver dysfunction contributes to ammonia intoxication by impairing the urea cycle, the primary mechanism for detoxifying ammonia. When the liver is damaged, it cannot effectively convert ammonia, a byproduct of protein metabolism, into urea for excretion. This leads to the accumulation of ammonia in the blood (hyperammonemia), which can cross the blood-brain barrier and cause neurotoxic effects such as cerebral edema, altered neurotransmitter balance, and neurological symptoms (e.g., confusion, lethargy, or coma). Conditions like hepatic encephalopathy are direct results of this impaired ammonia detoxification.
Question 9: What is the fundamental difference between transamination and deamination reactions in amino acid metabolism?
Answer: Transamination reactions involve the transfer of an amino group from an amino acid to an α-keto acid, typically α-ketoglutarate, forming a new amino acid and a new α-keto acid. Deamination, on the other hand, is the removal of an amino group from an amino acid, typically glutamate, releasing ammonia.
Question 10: What is the significance of glutamate in both transamination and deamination processes?
Answer: Glutamate plays a central role in amino acid metabolism, acting as a key intermediary in both transamination and deamination processes. Its significance lies in the following:
- In Transamination: Amino Group Transfer
- Glutamate as a Recipient: During transamination, glutamate serves as the main amino group acceptor. Aminotransferase enzymes transfer the amino group from other amino acids to α-ketoglutarate, forming glutamate. This allows for the safe collection of nitrogen from various amino acids.
- Glutamate as a Donor: Glutamate can also donate its amino group to other keto acids, regenerating α-ketoglutarate and synthesizing non-essential amino acids.
Example Reaction:
- Alanine + α-ketoglutarate ⇌ Pyruvate + Glutamate (catalyzed by alanine aminotransferase).
- In Deamination: Nitrogen Release
- Glutamate as a Nitrogen Carrier: Glutamate undergoes oxidative deamination via glutamate dehydrogenase to release its amino group as free ammonia (NH₃), which can then enter the urea cycle for detoxification in the liver.
- This process regenerates α-ketoglutarate, allowing it to participate in further transamination reactions.
Example Reaction:
- Glutamate + NAD⁺ (or NADP⁺) → α-Ketoglutarate + NH₃ + NADH (catalyzed by glutamate dehydrogenase).
- Central Role in Nitrogen Metabolism
- Nitrogen Collection: Glutamate collects nitrogen from various amino acids via transamination.
- Nitrogen Disposal: It releases nitrogen as ammonia during deamination, which is essential for excretion via the urea cycle.
Summary
Glutamate acts as a nitrogen shuttle in the body, linking the processes of transamination (amino group transfer) and deamination (amino group removal). This dual role makes it a key regulator of nitrogen metabolism and amino acid biosynthesis.
Question 11: Explain the importance of transamination reactions in amino acid biosynthesis.
Answer: Transamination reactions are essential for the synthesis of non-essential amino acids. By transferring an amino group from an available amino acid to an α-keto acid, the body can generate the required amino acids without relying solely on dietary intake.
Question 12: Describe the mechanism of a typical transamination reaction, including the role of the coenzyme involved.
Answer: A transamination reaction involves transferring an amino group from an amino acid to a keto acid, producing a new amino acid and keto acid. It is catalyzed by aminotransferase enzymes and requires the coenzyme pyridoxal phosphate (PLP), derived from vitamin B6.
Example:
- Alanine + α-ketoglutarate ⇌ Pyruvate + Glutamate
- Enzyme: Alanine aminotransferase (ALT).
This reaction is essential for amino acid biosynthesis, nitrogen metabolism, and energy pathways.
Question 13: How do deficiencies in certain vitamins impact transamination and amino acid metabolism?
Answer: Deficiencies in specific vitamins, particularly those required as coenzymes in amino acid metabolism, can severely impact transamination and related metabolic processes. Here’s how:
- Vitamin B6 (Pyridoxine) Deficiency
- Role in Transamination:
- Vitamin B6 is the precursor for pyridoxal phosphate (PLP), the essential coenzyme for all transamination reactions.
- PLP facilitates the transfer of amino groups between amino acids and keto acids.
- Impact of Deficiency:
- Impaired transamination disrupts amino acid metabolism, leading to an imbalance in amino acid pools.
- Accumulation of toxic nitrogenous compounds occurs due to impaired nitrogen transfer.
- Symptoms: Neurological issues (e.g., irritability, depression, seizures), microcytic anemia (due to reduced heme synthesis), and impaired protein metabolism.
- Vitamin B12 (Cobalamin) Deficiency
- Role in Amino Acid Metabolism:
- Vitamin B12 is required for the remethylation of homocysteine to methionine via methionine synthase.
- Impact of Deficiency:
- Elevated homocysteine levels (homocystinuria), which can lead to cardiovascular and neurological complications.
- Impaired synthesis of S-adenosylmethionine (SAM), a key methyl donor for various metabolic processes.
- Folic Acid (Vitamin B9) Deficiency
- Role in Amino Acid Metabolism:
- Folic acid is essential for the transfer of one-carbon units in amino acid metabolism, particularly in the conversion of serine to glycine and remethylation of homocysteine.
- Impact of Deficiency:
- Homocysteine accumulation, increasing the risk of cardiovascular disease.
- Impaired DNA synthesis and repair, affecting rapidly dividing cells.
- Symptoms: Macrocytic anemia and developmental delays.
- Niacin (Vitamin B3) Deficiency
- Role in Amino Acid Metabolism:
- Niacin (as NAD⁺/NADP⁺) is required for redox reactions in amino acid degradation pathways.
- Impact of Deficiency:
- Disruption of oxidative deamination reactions (e.g., by glutamate dehydrogenase).
- Symptoms: Pellagra (diarrhea, dermatitis, dementia).
Summary
Vitamin deficiencies disrupt critical coenzyme-dependent processes in amino acid metabolism, leading to metabolic imbalances, accumulation of toxic intermediates, and systemic symptoms like anemia, neurological issues, and cardiovascular risks. Supplementation is essential to restore normal metabolic functions.
Question 14: How do allosteric regulation and N-acetylglutamate contribute to the control of urea biosynthesis?
The urea cycle is tightly regulated to ensure the effective removal of excess nitrogen, primarily from amino acid metabolism. Allosteric regulation and N-acetylglutamate (NAG) play crucial roles in controlling this process:
- Allosteric Regulation of Carbamoyl Phosphate Synthetase I (CPS I)
- CPS I is the first and rate-limiting enzyme of the urea cycle.
- This mitochondrial enzyme catalyzes the formation of carbamoyl phosphate from ammonia (NH₃), bicarbonate (HCO₃⁻), and ATP.
- N-Acetylglutamate (NAG):
- Acts as an essential allosteric activator of CPS I.
- Without NAG, CPS I has little to no activity, even in the presence of its substrates.
- Role of N-Acetylglutamate (NAG)
- NAG Synthesis:
- Synthesized from glutamate and acetyl-CoA by the enzyme N-acetylglutamate synthase (NAGS).
- The production of NAG is stimulated by arginine, a key intermediate of the urea cycle, providing a feed-forward mechanism to enhance urea production.
- Mechanism of Action:
- NAG binds to CPS I and induces a conformational change, enhancing its affinity for substrates (ammonia and bicarbonate).
- This ensures that the urea cycle operates efficiently during periods of increased nitrogen load, such as after protein-rich meals.
- Regulatory Feedback Mechanisms
- Feed-forward Regulation:
- High levels of arginine stimulate NAG synthesis, which activates CPS I and drives the urea cycle.
- Substrate Availability:
- Increased levels of ammonia (e.g., during amino acid catabolism) promote urea cycle activity through substrate-driven stimulation of CPS I.
- Energy Availability:
- The urea cycle requires ATP. Energy depletion can indirectly limit the cycle.
- Pathological Implications
- NAGS Deficiency:
- Leads to reduced NAG levels, resulting in poor activation of CPS I and impaired urea cycle function.
- Causes hyperammonemia, which can manifest as lethargy, vomiting, and neurological deficits.
- Treated with carbamoyl glutamate, a synthetic analog of NAG that activates CPS I.
- CPS I Deficiency:
- Direct impairment of CPS I results in hyperammonemia due to ineffective ammonia detoxification.
Question 15: What are some common causes of hyperammonemia, and how do they lead to elevated ammonia levels?
Answer: Hyperammonemia, a condition characterized by elevated levels of ammonia in the blood, can result from a variety of causes, including:
Liver Dysfunction: The liver plays a crucial role in detoxifying ammonia through the urea cycle. When liver function is compromised, as in cases of cirrhosis, hepatitis, or liver failure, the liver cannot efficiently convert ammonia to urea for excretion. This leads to a buildup of ammonia in the bloodstream5.
Inherited Metabolic Disorders of the Urea Cycle: Genetic defects in any of the enzymes of the urea cycle or in the transport systems for urea cycle intermediates can lead to the accumulation of ammonia in the blood. These disorders are rare but can be serious, often presenting in infancy with symptoms of ammonia intoxication56.
Portal Systemic Shunting: If portal blood bypasses the liver, systemic blood ammonia may reach toxic levels. This can occur with severely impaired hepatic function or in the development of collateral links between the portal and systemic veins in cirrhosis5.
Reye’s Syndrome: This rare but serious condition typically affects children and is characterized by encephalopathy and liver dysfunction. The exact cause is unknown, but it is often associated with aspirin use during viral infections. The liver dysfunction in Reye’s syndrome can lead to hyperammonemia7.
These various causes of hyperammonemia all share the common feature of disrupting the normal detoxification of ammonia, leading to its accumulation in the blood. This accumulation can have toxic effects on the brain and other organs.
Question 16: Define uremia and explain the underlying causes of this condition.
Answer: Uremia, also known as uremic syndrome, is a clinical syndrome that develops when kidney function is severely impaired. It is characterized by the accumulation of urea and other nitrogenous waste products in the blood. Uremia is not only caused by the accumulation of urea itself, but also by the retention of other toxic metabolites that are normally cleared by the kidneys.
Causes of uremia can be classified as prerenal, renal, or postrenal, depending on where the underlying problem lies:
Prerenal: Factors that reduce blood flow to the kidneys, such as dehydration, heart failure, or shock, can cause prerenal uremia.
Renal: Damage to the kidneys themselves due to conditions like acute kidney injury, chronic kidney disease, glomerulonephritis, or polycystic kidney disease can result in renal uremia.
Postrenal: Obstruction of the urinary tract from causes like kidney stones, tumors, or an enlarged prostate can lead to postrenal uremia by preventing the flow of urine out of the body.
Regardless of the cause, uremia represents a serious condition that requires prompt medical attention.
Author:Namrata Chhabra
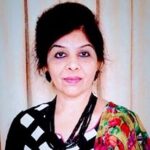