Library
“Clinical Biochemistry: Fatty Acid Metabolism, Ketogenesis, and Energy Regulation”
- November 23, 2024
- Posted by: Namrata Chhabra
- Category: Energy metabolism Library Metabolism of lipids Multiple-choice questions Multiple-Choice questions Practice questions Quizzes USMLE Content USMLE Style questions USMLE styled question bank
“Clinical Biochemistry Insights: Fatty Acid Metabolism and Energy Pathways”
1. In adipose tissue, through which of the following intermediates can glucose be converted to glycerol-3-phosphate for the esterification of fatty acids to form triglycerides?
A. Acetyl CoA
B. Dihydroxyacetone phosphate
C. Glycerol
D. Malate
E. Pyruvate
Correct Answer: B. Dihydroxyacetone phosphate:
Explanation: Dihydroxyacetone phosphate (DHAP), derived from glycolysis, is reduced to glycerol-3-phosphate by the enzyme glycerol-3-phosphate dehydrogenase.
Incorrect Options
A. Acetyl CoA: Incorrect. Acetyl CoA is involved in fatty acid synthesis and the TCA cycle, not directly in the conversion of glucose to glycerol-3-phosphate.
C. Glycerol: Incorrect. Adipose tissue lacks glycerol kinase, so glycerol cannot be directly converted to glycerol-3-phosphate.
D. Malate: Incorrect. Malate is part of the TCA cycle and gluconeogenesis but does not contribute directly to glycerol-3-phosphate synthesis.
E. Pyruvate: Incorrect. Pyruvate is primarily converted to acetyl CoA or oxaloacetate and does not play a direct role in glycerol-3-phosphate synthesis.
2. A 30-year-old woman has been fasting for religious reasons for the past 5 days. She complains of mild fatigue and occasional dizziness but is otherwise alert. A substance in her blood is now serving as an alternative energy source for her brain in response to prolonged fasting. Which of the following substances is most likely being utilized?
A. Acetone
B. Amino acids
C. Beta-hydroxybutyrate
D. Fatty acids
E. Glucose
Correct Answer: C. Beta-hydroxybutyrate
Explanations: In prolonged fasting and starvation, the brain shifts to utilizing beta-hydroxybutyrate, a ketone body, due to reduced glucose uptake and availability.
Incorrect Options
A. Acetone: Incorrect. Acetone is a byproduct of ketone body metabolism but is not utilized as an energy source by the brain.
B. Amino acids: Incorrect. Amino acids contribute to gluconeogenesis in the liver but are not directly used by the brain as an energy source.
D. Fatty acids: Incorrect. Fatty acids cannot cross the blood-brain barrier and are not used by the brain as an energy source.
E. Glucose: Partially correct. While glucose is still utilized, the brain relies heavily on ketone bodies (like beta-hydroxybutyrate) during conditions of high ketone production, such as starvation and Diabetic ketoacidosis.
3. A 3-month-old infant presents with vomiting, lethargy, and seizures following a mild viral illness. Laboratory tests reveal hypoglycemia without ketonuria. Which enzyme deficiency is most likely responsible for these findings?
A. Carnitine palmitoyltransferase I (CPT I) deficiency
B. Medium-chain acyl-CoA dehydrogenase (MCAD) deficiency
C. Acetyl-CoA carboxylase deficiency
D. Hormone-sensitive lipase deficiency
E. Fatty acid synthase deficiency
Correct Answer: B) Medium-chain acyl-CoA dehydrogenase (MCAD) deficiency
Explanation: MCAD deficiency disrupts the β-oxidation of medium-chain fatty acids, resulting in hypoketotic hypoglycemia during fasting or illness. The absence of ketones despite hypoglycemia is characteristic of this condition
Incorrect options
A) Carnitine palmitoyltransferase I (CPT I) deficiency: CPT I deficiency impairs the transport of long-chain fatty acids into mitochondria, leading to hypoketotic hypoglycemia. However, it typically presents with elevated plasma-free carnitine levels and more severe hepatic dysfunction.
C) Acetyl-CoA carboxylase deficiency: This deficiency affects fatty acid synthesis, leading to reduced fatty acid production. It is rare and typically presents with muscle weakness and developmental delays, not the acute symptoms described.
D) Hormone-sensitive lipase deficiency: Hormone-sensitive lipase is involved in mobilizing stored fats. Its deficiency would impair lipolysis, leading to fat accumulation, but it is not commonly associated with hypoglycemia or the acute presentation described.
E) Fatty acid synthase deficiency: Deficiency in fatty acid synthase affects the synthesis of fatty acids, leading to reduced fat stores and potential energy deficits. However, it is extremely rare and not typically associated with the acute symptoms described.
4. A 40-year-old morbidly obese man who had been consuming a carbohydrate-rich diet for several years has been advised to reduce his caloric intake, particularly carbohydrates. To achieve his weight loss goal, he begins a strict regimen involving prolonged fasting. As a result, his metabolic processes shift to limit fatty acid synthesis. Which of the following enzymes regulates fatty acid metabolism during both fed and fasting states?
A. Acetyl-CoA carboxylase
B. Acetyl transacylase
C. ATP citrate lyase
D. Thioesterase
E. Thiolase
Correct Answer: A. Acetyl-CoA carboxylase.
Explanation: Acetyl-CoA carboxylase (ACC) is the key regulatory enzyme in de novo fatty acid synthesis. It catalyzes the conversion of acetyl-CoA to malonyl-CoA, the rate-limiting step in fatty acid synthesis. ACC is activated in the fed state (via insulin) and inhibited during fasting or energy demand (via AMP-activated protein kinase).
Incorrect options
B. Acetyl transacylase: Incorrect. Acetyl transacylase is part of the fatty acid synthase complex, facilitating the attachment of acetyl-CoA to the enzyme, but does not regulate fatty acid synthesis.
C. ATP citrate lyase: Incorrect. ATP citrate lyase converts citrate to acetyl-CoA in the cytoplasm, providing a substrate for fatty acid synthesis. However, it is not the regulatory enzyme controlling fatty acid synthesis.
D. Thioesterase: Incorrect. Thioesterase is part of the fatty acid synthase complex, responsible for releasing the final fatty acid product (e.g., palmitate), but does not regulate the process.
E. Thiolase: Incorrect. Thiolase is involved in β-oxidation (fatty acid breakdown) and ketone body synthesis but has no role in regulating fatty acid synthesis.
5. A 45-year-old male with a history of poorly controlled diabetes presents to the emergency room with confusion and deep, rapid breathing. Blood tests reveal significantly elevated blood glucose and ketone levels. His anion gap is high, and serum bicarbonate is low, indicating metabolic acidosis. The physician suspects diabetic ketoacidosis (DKA). Which of the following substances is the primary energy source for his red blood cells in this condition?
A. Acetone
B. Amino acids
C. Beta-hydroxybutyrate
D. Fatty acids
E. Glucose
Correct Answer: E. Glucose:
Explanation: Despite hyperglycemia in DKA, glucose remains the primary energy source for red blood cells, as glycolysis occurs in the cytoplasm and does not require mitochondria.
Incorrect options
A. Acetone: Incorrect. Acetone is a byproduct of ketone body metabolism but cannot be utilized as an energy source by red blood cells.
B. Amino acids: Incorrect. Amino acids are utilized by the liver for gluconeogenesis but are not directly used by red blood cells for energy.
C. Beta-hydroxybutyrate: Incorrect. Beta-hydroxybutyrate is a ketone body used by tissues such as the brain and muscles during starvation or DKA. However, red blood cells lack mitochondria and cannot oxidize ketone bodies.
D. Fatty acids: Incorrect. Fatty acids require mitochondrial oxidation for energy production, but red blood cells lack mitochondria and cannot utilize fatty acids.
6. A newborn presents with hypoketotic hypoglycemia. Which enzyme deficiency is most likely?
A. Medium-chain acyl-CoA dehydrogenase (MCAD)
B. Carnitine palmitoyltransferase I
C. Glucose-6-phosphatase
D. Hormone-sensitive lipase
E. ATP citrate lyase
Correct Answer: A. Medium-chain acyl-CoA dehydrogenase (MCAD)
Explanation:
• MCAD deficiency is one of the most common inborn errors of metabolism, affecting the β-oxidation of medium-chain fatty acids (6–12 carbons) in the mitochondria.
• Normally, β-oxidation breaks down fatty acids to acetyl-CoA, which enters the TCA cycle for energy production and contributes to ketogenesis during fasting.
• In MCAD deficiency, the inability to oxidize medium-chain fatty acids results in a lack of ketone body production (hypoketosis) and a severe energy deficit during fasting or periods of increased energy demand (e.g., illness).
• This leads to hypoglycemia because glucose becomes the sole energy source, and glycogen stores are rapidly depleted.
• Clinical signs often present in infancy or early childhood and include lethargy, vomiting, seizures, and hypoketotic hypoglycemia after prolonged fasting.
• Diagnosis is confirmed by detecting elevated levels of medium-chain acylcarnitines in the blood or urine.
Incorrect Options:
B. Carnitine palmitoyltransferase I (CPT1):
o CPT1 deficiency prevents the transport of long-chain fatty acids into the mitochondria for β-oxidation.
o It also results in hypoketotic hypoglycemia, as long-chain fatty acids cannot contribute to ketone production.
o However, MCAD deficiency is much more common in the younger age group and affects a broader range of fatty acid oxidation intermediates, making it the more likely diagnosis in this scenario.
C. Glucose-6-phosphatase:
o Glucose-6-phosphatase is involved in gluconeogenesis and glycogenolysis (converting glucose-6-phosphate into free glucose).
o Its deficiency causes glycogen storage disease type I (von Gierke disease), characterized by fasting hypoglycemia, hepatomegaly, and lactic acidosis, but does not result in hypoketosis.
o Ketogenesis remains intact because fatty acid oxidation pathways are unaffected.
D. Hormone-sensitive lipase (HSL):
o HSL mobilizes stored triglycerides in adipose tissue by hydrolyzing them into free fatty acids and glycerol.
o A deficiency would reduce the availability of free fatty acids for β-oxidation but does not directly impair mitochondrial fatty acid metabolism.
o HSL deficiency is rare and does not typically cause hypoketotic hypoglycemia.
E. ATP citrate lyase:
o ATP citrate lyase is part of fatty acid synthesis, not fatty acid oxidation.
o It converts citrate into acetyl-CoA in the cytoplasm during the fed state for lipogenesis.
o This enzyme is not involved in fasting metabolism, ketogenesis, or hypoglycemia.
Key Learning:
• MCAD deficiency leads to hypoketotic hypoglycemia due to a failure in β-oxidation of medium-chain fatty acids.
• During fasting, energy demands shift to fat oxidation and ketogenesis. A defect in this pathway results in severe energy deficits.
• Other conditions like CPT1 deficiency or gluconeogenesis defects may mimic some symptoms but can be differentiated based on the metabolic pathways involved.
7. During exercise, which enzyme activates fatty acid oxidation by reducing malonyl-CoA levels?
A. AMP-activated protein kinase (AMPK)
B. Acetyl-CoA carboxylase (ACC)
C. Lipoprotein lipase (LPL)
D. Hormone-sensitive lipase (HSL)
E. Fatty acid synthase (FAS)
Correct Answer: A. AMP-activated protein kinase (AMPK)
Explanation:
• AMPK phosphorylates and inhibits acetyl-CoA carboxylase (ACC), the enzyme responsible for converting acetyl-CoA to malonyl-CoA.
• Malonyl-CoA is a potent inhibitor of carnitine palmitoyltransferase I (CPT1), the enzyme that transports long-chain fatty acids into mitochondria for β-oxidation.
• By reducing malonyl-CoA levels, AMPK removes this inhibition, allowing fatty acids to enter mitochondria and undergo oxidation, providing an alternative energy source during exercise.
• This regulation ensures that energy is efficiently derived from fat stores during prolonged exercise or energy depletion.
Incorrect Options:
B. Acetyl-CoA carboxylase (ACC):
o ACC is the enzyme that synthesizes malonyl-CoA from acetyl-CoA, promoting fatty acid synthesis in the cytoplasm.
o During exercise, ACC activity is inhibited by AMPK to favor β-oxidation.
o Therefore, ACC is not involved in the activation of fatty acid oxidation and does the opposite by supporting synthesis.
2. C. Lipoprotein lipase (LPL):
o LPL is an enzyme that hydrolyzes triglycerides in circulating lipoproteins (e.g., chylomicrons and VLDL) into free fatty acids and glycerol, allowing uptake by tissues.
o LPL activity facilitates the availability of fatty acids for energy use but does not directly regulate mitochondrial fatty acid oxidation or malonyl-CoA levels.
D. Hormone-sensitive lipase (HSL):
o HSL hydrolyzes stored triglycerides in adipose tissue into free fatty acids and glycerol.
o While HSL increases the availability of fatty acids during exercise, it does not influence malonyl-CoA levels or mitochondrial fatty acid transport.
o Its role is primarily in lipolysis, not the regulation of oxidation.
E. Fatty acid synthase (FAS):
o FAS is involved in de novo fatty acid synthesis, catalyzing the elongation of fatty acid chains in the cytoplasm.
o During exercise, fatty acid synthesis is downregulated, and FAS activity is suppressed, making it irrelevant to β-oxidation.
8. A researcher is studying the pathway of ketolysis to enhance energy utilization in obese individuals on a ketogenic diet. Which of the following is an intermediate of ketolysis that provides energy to peripheral tissues?
A. Acetoacetyl-CoA
B. Fatty acyl-CoA
C. HMG-CoA
D. Propionyl-CoA
E. Succinyl-CoA
Correct Answer: A. Acetoacetyl-CoA
Explanation:
• In ketolysis, acetoacetate is converted into acetoacetyl-CoA, which is further broken down into acetyl-CoA by the enzyme thiolase.
• Acetyl-CoA enters the TCA cycle to produce ATP, providing energy for peripheral tissues like muscles and the brain.
Incorrect Options:
B. Fatty acyl-CoA: Involved in β-oxidation of fatty acids, not ketolysis.
C. HMG-CoA: An intermediate in ketogenesis (ketone body production in the liver), not ketolysis.
D. Propionyl-CoA: A product of odd-chain fatty acid metabolism unrelated to ketolysis.
E. Succinyl-CoA: Used to activate acetoacetate in ketolysis but is not directly metabolized for energy.
9. In the fed state, excess citrate is transported to the cytoplasm. Which enzyme converts citrate to acetyl-CoA?
A. ATP citrate lyase
B. Acetyl-CoA carboxylase
C. Fatty acid synthase
D. Malic enzyme
E. Carnitine palmitoyltransferase I
Correct Answer: A. ATP citrate lyase
Explanation:
• ATP citrate lyase cleaves citrate (exported from the mitochondria) into acetyl-CoA and oxaloacetate in the cytoplasm.
• Acetyl-CoA is used for fatty acid synthesis, while oxaloacetate is recycled back to the mitochondria via malate or pyruvate.
Incorrect Options:
B. Acetyl-CoA carboxylase:
o Catalyzes the conversion of acetyl-CoA to malonyl-CoA, the first committed step in fatty acid synthesis.
o However, it does not convert citrate to acetyl-CoA.
C. Fatty acid synthase:
o A multifunctional enzyme that elongates fatty acid chains by adding 2-carbon units from malonyl-CoA.
o It uses acetyl-CoA but does not generate it.
D. Malic enzyme:
o Converts malate to pyruvate in the cytoplasm, generating NADPH required for fatty acid synthesis.
o It does not directly act on citrate.
E. Carnitine palmitoyltransferase I (CPT1):
o Regulates the transport of long-chain fatty acids into mitochondria for β-oxidation.
o It has no role in citrate metabolism or fatty acid synthesis.
10. A 24-year-old woman presents to the emergency department with nausea, abdominal pain, and confusion. She has a history of type 1 diabetes and reports missing her insulin doses over the past three days. On examination, she is tachypneic with deep, rapid breathing (Kussmaul respiration). Blood tests reveal elevated blood glucose, high anion gap metabolic acidosis, and significantly increased serum ketones. During this state, which of the following tissues cannot utilize ketone bodies for energy?
A. Brain
B. Heart
C. Skeletal muscle
D. Red blood cells
E. Kidney
Correct Answer: D. Red blood cells
Explanation:
• Red blood cells (RBCs) lack mitochondria, which are essential for ketone body metabolism.
• Mitochondria are required to oxidize ketone bodies like acetoacetate and beta-hydroxybutyrate via the TCA cycle. Without mitochondria, RBCs rely exclusively on glycolysis for ATP production.
Incorrect Options:
A. Brain:
o During severe metabolic states like diabetic ketoacidosis (DKA), the brain uses ketone bodies (particularly beta-hydroxybutyrate) for energy, reducing its dependence on glucose.
B. Heart:
o The heart is highly efficient at oxidizing ketone bodies, especially during states of prolonged fasting, starvation, or DKA, as they are a preferred energy source.
C. Skeletal muscle:
o Skeletal muscles readily oxidize ketone bodies for ATP production during fasting or DKA, conserving glucose for glucose-dependent tissues like RBCs.
E. Kidney:
o The renal cortex efficiently utilizes ketone bodies as an energy source during ketoacidosis, contributing to energy conservation in fasting states.
Clinical Context and Key Takeaway:
In diabetic ketoacidosis (DKA), ketone bodies serve as an alternative energy source for many tissues, sparing glucose for critical cells like RBCs and nervous tissue. However, RBCs cannot utilize ketones due to the absence of mitochondria, relying solely on glycolysis for ATP.
11. A 60-year-old man with poorly controlled diabetes presents with fatigue and difficulty managing his weight. Lab tests reveal elevated blood triglyceride levels. During fasting, which enzyme in adipose tissue is responsible for hydrolyzing triglycerides to release free fatty acids for energy?
A. Lipoprotein lipase
B. Hormone-sensitive lipase
C. Pancreatic lipase
D. Acetyl-CoA carboxylase
E. Fatty acid synthase
Correct Answer: B. Hormone-sensitive lipase
Explanation:
• Hormone-sensitive lipase (HSL) is activated during fasting in response to low insulin and high glucagon/epinephrine levels.
• It hydrolyzes stored triglycerides in adipose tissue into free fatty acids and glycerol, which are then released into the bloodstream.
• Free fatty acids are transported to peripheral tissues for β-oxidation, while glycerol is used in gluconeogenesis by the liver.
Incorrect Options:
A. Lipoprotein lipase:
o Lipoprotein lipase acts on circulating triglycerides in chylomicrons and very low-density lipoproteins (VLDL), releasing free fatty acids for uptake by tissues.
o It is not involved in mobilizing stored triglycerides from adipose tissue.
C. Pancreatic lipase:
o Pancreatic lipase digests dietary triglycerides in the intestine, breaking them down into monoglycerides and free fatty acids for absorption.
o It is unrelated to fasting metabolism or adipose tissue triglyceride breakdown.
D. Acetyl-CoA carboxylase:
o Acetyl-CoA carboxylase is involved in fatty acid synthesis by converting acetyl-CoA to malonyl-CoA.
o It is active during the fed state, not fasting, and does not hydrolyze triglycerides.
E. Fatty acid synthase:
o Fatty acid synthase catalyzes the elongation of fatty acid chains during de novo lipogenesis.
o It is also active in the fed state and does not play a role in triglyceride hydrolysis.
Key Takeaway:
During fasting, hormone-sensitive lipase (HSL) is the primary enzyme responsible for breaking down stored triglycerides in adipose tissue, providing free fatty acids and glycerol for energy production. Other enzymes play roles in dietary fat digestion or fatty acid synthesis but are not involved in this process.
12. After the Christmas party, a young lady who is extremely weight-conscious feels stressed about overindulging in food. Which of the following processes is expected to occur in the well-fed state?
A. Conversion of pyruvate to oxaloacetate
B. Decreased activity of acetyl-CoA carboxylase
C. Decreased activity of hormone-sensitive lipase
D. Mitochondrial fatty acid oxidation
E. Transport of glucose into liver cells
Correct Answer: C. Decreased activity of hormone-sensitive lipase
Explanation:
• In the well-fed state, high insulin levels inhibit hormone-sensitive lipase (HSL) in adipose tissue.
• HSL is responsible for breaking down stored triglycerides into free fatty acids and glycerol during fasting or low insulin states.
• By inhibiting HSL, insulin prevents unnecessary lipolysis, promoting fat storage instead of mobilization.
Incorrect Options:
A. Conversion of pyruvate to oxaloacetate:
o This occurs during gluconeogenesis, which is suppressed in the well-fed state. Pyruvate is more likely converted to acetyl-CoA for fatty acid or energy production in this context.
B. Decreased activity of acetyl-CoA carboxylase:
o Acetyl-CoA carboxylase activity increases in the well-fed state due to insulin. It facilitates fatty acid synthesis by producing malonyl-CoA.
D. Mitochondrial fatty acid oxidation:
o Fatty acid oxidation is inhibited in the well-fed state because malonyl-CoA (produced by acetyl-CoA carboxylase) blocks carnitine palmitoyltransferase I (CPT1), preventing fatty acid transport into mitochondria.
E. Transport of glucose into liver cells:
o Glucose enters liver cells via GLUT2 transporters, which are not insulin-dependent. Therefore, this process is unaffected by the well-fed or fasting state.
Key Takeaway:
In the well-fed state, insulin suppresses fat breakdown by decreasing hormone-sensitive lipase (HSL) activity, favoring energy storage as fat and glycogen. GLUT2 transporters in the liver are not under insulin control, making option C the correct answer.
13. Which set of molecules is directly produced by β-oxidation of fatty acids?
A. NADH and FADH₂
B. Malonyl-CoA and Acetyl-CoA
C. Acetoacetate and Beta-hydroxybutyrate
D. Glycerol and Free Fatty Acids
E. Glucose and Pyruvate
Correct Answer: A. NADH and FADH₂
Explanation:
• During β-oxidation, fatty acids are broken down in the mitochondria into acetyl-CoA.
• Each cycle of β-oxidation produces NADH and FADH₂ during specific oxidative steps, which are later used in the electron transport chain (ETC) to generate ATP.
Incorrect Options:
B. Malonyl-CoA and Acetyl-CoA:
o Malonyl-CoA is involved in fatty acid synthesis, not oxidation.
o Acetyl-CoA is produced during β-oxidation, but it is not paired with malonyl-CoA in this pathway.
C. Acetoacetate and Beta-hydroxybutyrate:
o These are ketone bodies, produced in the liver from acetyl-CoA, which is indirectly derived from β-oxidation.
o They are not direct products of the β-oxidation pathway itself.
D. Glycerol and Free Fatty Acids:
o These are products of triglyceride hydrolysis in adipose tissue, not β-oxidation.
o Free fatty acids enter β-oxidation, but they are not produced by it.
E. Glucose and Pyruvate:
o Glucose and pyruvate are part of glycolysis and carbohydrate metabolism, not fatty acid oxidation.
Key Takeaway:
NADH and FADH₂ are directly produced during the oxidative steps of β-oxidation, which fuel the electron transport chain for ATP generation. Other options involve distinct pathways or secondary products.
14. Laboratory tests of a 3-month-old child reveal elevated levels of very long-chain fatty acids (VLCFAs) and phytanic acid in the plasma. Which of the following organelles is most likely affected in this infant?
A. Nucleus
B. Rough endoplasmic reticulum
C. Lysosomes
D. Mitochondria
E. Peroxisomes
Correct Answer: E. Peroxisomes
Explanation:
• Peroxisomes are essential for the metabolism of very long-chain fatty acids (VLCFAs) and branched-chain fatty acids, such as phytanic acid, through a process called β-oxidation and α-oxidation, respectively.
• Defects in peroxisomal function or biogenesis, such as in Zellweger syndrome or related peroxisomal disorders, lead to the accumulation of VLCFAs and phytanic acid in the blood and tissues.
• Clinical symptoms often include developmental delay, hypotonia, seizures, hepatomegaly, and dysmorphic features.
Incorrect Options:
A. Nucleus:
o The nucleus is primarily involved in the storage and transcription of genetic material (DNA and RNA).
o It has no role in the metabolism of VLCFAs or phytanic acid.
B. Rough endoplasmic reticulum (RER):
o The RER is involved in protein synthesis, folding, and post-translational modifications.
o It does not participate in fatty acid metabolism or degradation.
C. Lysosomes:
o Lysosomes are responsible for the degradation of macromolecules like proteins, lipids, and carbohydrates via hydrolytic enzymes.
o While lysosomal storage diseases affect lipid metabolism, they do not involve VLCFAs or phytanic acid.
D. Mitochondria:
o Mitochondria are responsible for the β-oxidation of short- and medium-chain fatty acids, not VLCFAs.
o VLCFAs must first undergo initial oxidation in the peroxisomes before being shortened and transferred to the mitochondria for further metabolism.
Key Takeaway:
The peroxisomes are the organelles responsible for the initial oxidation of very long-chain and branched-chain fatty acids. Defects in peroxisomal function result in the accumulation of VLCFAs and phytanic acid, as seen in disorders like Zellweger syndrome.
15. During fasting, free fatty acids are converted to ketone bodies in the liver. What is the primary ketone body formed?
A. Beta-hydroxybutyrate
B. Acetone
C. Malonyl-CoA
D. Acetoacetate
E. Oxaloacetate
Correct Answer: D. Acetoacetate
Explanation:
• Acetoacetate is the first ketone body formed during ketogenesis in the liver.
• It is synthesized from acetyl-CoA via HMG-CoA synthase and HMG-CoA lyase.
• Acetoacetate can either:
1. Be reduced to beta-hydroxybutyrate in a reaction catalyzed by beta-hydroxybutyrate dehydrogenase (dependent on the NADH/NAD⁺ ratio).
2. Undergo spontaneous decarboxylation to form acetone, which is excreted or volatilized.
Incorrect Options:
A. Beta-hydroxybutyrate:
o Although beta-hydroxybutyrate becomes the predominant ketone body in circulation, it is formed by the reduction of acetoacetate and is not the first ketone body produced in the liver.
B. Acetone:
o Acetone is a byproduct of acetoacetate decarboxylation and is not metabolized for energy. It plays a minor role in ketone body metabolism.
C. Malonyl-CoA:
o Malonyl-CoA is a key intermediate in fatty acid synthesis and inhibits fatty acid oxidation by suppressing CPT1. It has no role in ketogenesis.
E. Oxaloacetate:
o Oxaloacetate is a substrate for gluconeogenesis. During fasting, its depletion in the liver shifts acetyl-CoA toward ketogenesis instead of the TCA cycle.
Key Takeaway:
Acetoacetate is the first ketone body formed during ketogenesis and serves as the precursor for both beta-hydroxybutyrate and acetone, making it the primary ketone body produced in the liver.
16. A two-month-old child is brought in an unconscious state in the pediatric emergency department. Her blood glucose level is 45 mg/d, and further blood chemistry reveals very low levels of ketone bodies, normal levels of carnitine and acyl-carnitine, but elevated levels of dicarboxylic acids. Which of the following enzymes is most likely defective in this child?
A. Carnitine acyltransferase I
B. Carnitine acyltransferase II
C. Fatty acid synthase complex
D. Acetyl CoA carboxylase
E. Medium-chain acyl-CoA dehydrogenase
Correct Answer: E. Medium-chain acyl-CoA dehydrogenase (MCAD)
Explanation:
• Medium-chain acyl-CoA dehydrogenase (MCAD) is an essential enzyme in β-oxidation, specifically for oxidizing medium-chain fatty acids (6–12 carbons) in the mitochondria.
• Deficiency of MCAD leads to:
1. Impaired fatty acid oxidation.
2. Reduced ketone body production, as acetyl-CoA derived from β-oxidation, is a precursor for ketogenesis.
3. Hypoglycemia, as fatty acid oxidation normally provides energy to spare glucose during fasting.
4. Accumulation of dicarboxylic acids, which are formed via ω-oxidation, a compensatory pathway for fatty acid metabolism.
• Normal carnitine and acyl-carnitine levels indicate that the defect is beyond the transport of fatty acids into the mitochondria, further supporting MCAD deficiency.
Incorrect Options:
A. Carnitine acyltransferase I (CPT1):
o CPT1 deficiency leads to impaired transport of long-chain fatty acids into the mitochondria, resulting in low ketone levels and hypoglycemia.
o However, CPT1 deficiency is associated with low acyl-carnitine levels, not normal levels as seen in this case.
B. Carnitine acyltransferase II (CPT2):
o CPT2 deficiency also affects long-chain fatty acid oxidation and leads to hypoketotic hypoglycemia.
o It is typically associated with elevated acyl-carnitine levels, which are absent in this case.
C. Fatty acid synthase complex:
o This enzyme is involved in fatty acid synthesis, not oxidation.
o Defects in fatty acid synthesis do not result in hypoglycemia or elevated dicarboxylic acids.
D. Acetyl CoA carboxylase:
o Acetyl-CoA carboxylase is involved in the synthesis of malonyl-CoA, a regulator of fatty acid synthesis.
o Its deficiency does not lead to hypoketotic hypoglycemia or elevated dicarboxylic acids.
Key Takeaway:
MCAD deficiency is the most likely diagnosis in a child with hypoketotic hypoglycemia, normal acyl-carnitine levels, and elevated dicarboxylic acids. This condition impairs fatty acid oxidation, leading to energy deficits during fasting. Early diagnosis and management with frequent feeding and avoidance of fasting are crucial to prevent severe complications.
17. In the fed state, insulin promotes fatty acid synthesis by activating which enzyme?
A. Hormone-sensitive lipase
B. Acetyl-CoA carboxylase
C. Carnitine palmitoyltransferase II
D. Glycogen phosphorylase
E. Thiolase
Correct Answer: B. Acetyl-CoA carboxylase
Explanation:
• Insulin activates Acetyl-CoA carboxylase (ACC) through dephosphorylation via protein phosphatase activation.
• ACC catalyzes the rate-limiting step of fatty acid synthesis: the conversion of acetyl-CoA to malonyl-CoA.
• Malonyl-CoA is a key precursor for fatty acid elongation and also inhibits Carnitine palmitoyltransferase I (CPT1) to suppress fatty acid oxidation, ensuring fatty acids are synthesized and stored during the fed state.
Incorrect Options:
A. Hormone-sensitive lipase:
o HSL is active during fasting, not the fed state.
o Insulin inhibits HSL, reducing the release of free fatty acids from stored triglycerides.
C. Carnitine palmitoyltransferase II (CPT2):
o CPT2 is involved in mitochondrial fatty acid oxidation by facilitating the transport of acyl-carnitine derivatives into the mitochondria.
o This process is suppressed in the fed state due to high levels of malonyl-CoA.
D. Glycogen phosphorylase:
o Glycogen phosphorylase breaks down glycogen to release glucose, a process that is inhibited in the fed state by insulin.
E. Thiolase:
o Thiolase catalyzes the cleavage of acyl-CoA into acetyl-CoA during β-oxidation, a pathway that is suppressed in the fed state.
Key Takeaway:
In the fed state, insulin promotes fatty acid synthesis by activating Acetyl-CoA carboxylase (ACC), ensuring energy is stored as fat rather than oxidized. Other enzymes listed are involved in processes more active during fasting or energy deficit states.
18. A pharmaceutical company is developing an anti-obesity drug that promotes fatty acid oxidation by upregulating an enzyme that initiates fatty acid oxidation. This enzyme activates fatty acids by converting them into an active intermediate, facilitating their entry into the metabolic pathway. Which of the following enzymes might be the potential target?
A. Acetyl-CoA carboxylase
B. Carnitine palmitoyltransferase I (CPT1)
C. Fatty acyl-CoA synthetase (Acyl-CoA synthetase)
D. Fatty acid synthase
E. Hormone-sensitive lipase
Correct Answer: C. Fatty acyl-CoA synthetase (Acyl-CoA synthetase)
Explanation:
• Fatty acyl-CoA synthetase (also known as acyl-CoA synthetase) is the enzyme responsible for the activation of free fatty acids.
• It catalyzes the conversion of a fatty acid into fatty acyl-CoA, an active intermediate required for entry into subsequent pathways like β-oxidation.
• This activation is essential for fatty acids to be transported into mitochondria for energy production.
Incorrect Options:
A. Acetyl-CoA carboxylase (ACC):
o ACC is involved in fatty acid synthesis, not oxidation. It catalyzes the conversion of acetyl-CoA to malonyl-CoA, a precursor for fatty acid synthesis.
o Malonyl-CoA inhibits CPT1, which prevents fatty acid oxidation.
B. Carnitine palmitoyltransferase I (CPT1):
o CPT1 is crucial for the transport of long-chain fatty acids into the mitochondria by converting fatty acyl-CoA into fatty acyl-carnitine.
o However, it does not initiate fatty acid oxidation; it facilitates a downstream step.
D. Fatty acid synthase:
o Fatty acid synthase catalyzes the elongation of fatty acid chains during de novo synthesis, not fatty acid oxidation.
E. Hormone-sensitive lipase:
o Hormone-sensitive lipase is involved in lipolysis, the hydrolysis of triglycerides into free fatty acids and glycerol.
o It does not play a direct role in activating fatty acids for oxidation.
Key Takeaway:
The enzyme fatty acyl-CoA synthetase (Acyl-CoA synthetase) initiates fatty acid oxidation by converting free fatty acids into their active form, fatty acyl-CoA, making it the ideal target for a drug promoting fatty acid oxidation.
19. A 35-year-old woman with type 2 diabetes comes to the clinic for follow-up. She reports unintended weight loss of 5 kg over the past month. Her physician starts her on insulin therapy to improve glycemic control. She wants to understand how insulin will regulate fat metabolism and help prevent further weight loss. Which of the following mechanisms explains how insulin downregulates lipolysis in adipose tissue?
A. Allosteric modification of hormone-sensitive lipase
B. Increasing the activity of phosphatase
C. Increasing the concentration of cAMP
D. Increasing the activation of protein kinase A
E. Repressing the expression of the hormone-sensitive lipase gene
Correct Answer: B. Increasing the activity of phosphatase
Explanation:
• Insulin decreases fat breakdown in adipose tissue by activating protein phosphatases, which dephosphorylate and inactivate hormone-sensitive lipase (HSL).
• Dephosphorylated HSL is inactive, reducing the breakdown of triglycerides into free fatty acids and glycerol, thereby limiting lipolysis.
• Additionally, insulin lowers cAMP levels by activating phosphodiesterase, suppressing protein kinase A (PKA) activity, which further reduces HSL phosphorylation and activity.
Incorrect Options:
A. Allosteric modification of hormone-sensitive lipase:
o Insulin does not regulate HSL through allosteric modification. HSL activity is controlled through phosphorylation (activation) and dephosphorylation (inactivation).
C. Increasing the concentration of cAMP:
o Insulin decreases, not increases, cAMP levels by activating phosphodiesterase, which hydrolyzes cAMP into AMP. Elevated cAMP promotes lipolysis, contrary to insulin’s effects.
D. Increasing the activation of protein kinase A:
o Protein kinase A (PKA) is activated by cAMP and phosphorylates HSL, promoting lipolysis. Insulin inhibits this process by lowering cAMP levels.
E. Repressing the expression of the hormone-sensitive lipase gene:
o Insulin regulates lipolysis at the post-translational level, not by repressing HSL gene expression.
Key Takeaway:
Insulin prevents excessive fat breakdown by activating protein phosphatases, dephosphorylating and inactivating hormone-sensitive lipase, and reducing cAMP levels. This helps limit unintended weight loss by preserving fat stores in adipose tissue.
20. A pharmaceutical researcher is studying how insulin regulates acetyl-CoA carboxylase (ACC), a key enzyme in fatty acid synthesis. Insulin exerts both short-term and long-term effects on ACC. Which of the following mechanisms best explains how insulin regulates ACC activity?
A. Allosteric and covalent modification
B. Allosteric modification and induction
C. Covalent modification and induction
D. Feedback inhibition
E. Feedforward regulation
Correct Answer: C. Covalent modification and induction
Explanation:
Insulin regulates acetyl-CoA carboxylase (ACC) through short-term and long-term mechanisms:
1. Short-term Regulation (Covalent Modification):
o Insulin activates protein phosphatase 2A (PP2A), which dephosphorylates and activates ACC.
o This enhances ACC’s enzymatic activity, promoting the conversion of acetyl-CoA to malonyl-CoA and initiating fatty acid synthesis.
o This rapid response allows cells to adjust metabolism immediately in response to the fed state.
2. Long-term Regulation (Induction):
o Insulin increases the transcription of the ACC gene, leading to enhanced synthesis of the ACC enzyme.
o This ensures a sustained increase in fatty acid synthesis during prolonged feeding.
These mechanisms work together to promote fatty acid synthesis in energy-rich states.
Incorrect Options:
A. Allosteric and covalent modification:
o Covalent modification (via dephosphorylation) is a valid mechanism, but allosteric regulation of ACC by citrate is not directly mediated by insulin.
B. Allosteric modification and induction:
o Induction of ACC occurs due to insulin, but allosteric modification (e.g., activation by citrate) is not insulin’s primary mode of regulation.
D. Feedback inhibition:
o ACC activity is not regulated by feedback inhibition. Instead, malonyl-CoA inhibits fatty acid oxidation by suppressing CPT1, a separate process.
E. Feedforward regulation:
o Feedforward regulation implies that insulin would act indirectly to promote fatty acid synthesis, but insulin directly modifies ACC activity via covalent changes and gene induction.
21. During the complete oxidation of a fatty acid with 15 carbon atoms, which of the following is/are produced?
A. Acetyl CoA
B. Butyryl CoA
C. Propionyl CoA
D. Both Acetyl CoA and Butyryl CoA
E. Both Butyryl CoA and Propionyl CoA
F. Both Acetyl CoA and Propionyl CoA
Correct Answer: F. Both Acetyl CoA and Propionyl CoA
Explanation:
• A 15-carbon fatty acid is an odd-chain fatty acid, which undergoes β-oxidation in the mitochondria.
• β-oxidation removes 2-carbon units as acetyl CoA in each cycle. However, at the final step of oxidation for odd-chain fatty acids:
o Propionyl CoA (a 3-carbon compound) is produced, along with acetyl CoA from the previous steps.
Incorrect Options:
A. Acetyl CoA:
o While acetyl CoA is produced during β-oxidation, it is not the only product in odd-chain fatty acid oxidation. Propionyl CoA is also formed in the final step.
B. Butyryl CoA:
o Butyryl CoA is not a product of β-oxidation. It is an intermediate in fatty acid elongation and does not appear during oxidation.
C. Propionyl CoA:
o Propionyl CoA is produced, but it is not the only product. Acetyl CoA is the primary product of β-oxidation.
D. Both Acetyl CoA and Butyryl CoA:
o Butyryl CoA is not involved in β-oxidation, making this combination incorrect.
E. Both Butyryl CoA and Propionyl CoA:
o Propionyl CoA is correct, but butyryl CoA is not a product of β-oxidation, so this combination is invalid.
Key Takeaway:
The complete oxidation of a 15-carbon odd-chain fatty acid produces 6 acetyl CoA molecules and 1 propionyl CoA, making both acetyl CoA and propionyl CoA the correct answer.
22. When stearic acid is completely oxidized, how many acetyl-CoA molecules are produced?
A. 1
B. 8
C. 9
D. 2
E. 10
Correct Answer: C. 9
Explanation:
• Stearic acid is a saturated fatty acid with 18 carbon atoms.
• During β-oxidation, each cycle removes 2 carbons in the form of acetyl-CoA, until the entire fatty acid is oxidized.
• The total number of acetyl-CoA molecules produced is determined by dividing the total carbon count by 2
• Thus, the complete oxidation of stearic acid yields 9 acetyl-CoA molecules, which enter the TCA cycle for further energy production.
Incorrect Options:
A. 1:
o This is incorrect, as a single acetyl-CoA is produced per β-oxidation cycle, but stearic acid undergoes multiple cycles.
B. 8:
o This is incorrect, as stearic acid with 18 carbons produces 9 acetyl-CoA molecules, not 8.
D. 2:
o Only short-chain fatty acids like butyric acid (4 carbons) produce 2 acetyl-CoA molecules. Stearic acid has far more carbons.
E. 10:
o This is incorrect, as stearic acid has 18 carbons, and the number of acetyl-CoA produced is 9, not 10.
Key Takeaway:
The complete oxidation of stearic acid (18 carbons) through β-oxidation produces 9 acetyl-CoA molecules, each of which enters the TCA cycle for further ATP generation.
23. An infant is diagnosed with Zellweger syndrome, a rare genetic disorder. Which metabolic pathway is primarily affected in this condition?
A. Peroxisomal oxidation
B. Alpha oxidation
C. Beta oxidation
D. Omega oxidation
E. Ketogenesis
Correct Answer: A. Peroxisomal oxidation
Explanation:
• Zellweger syndrome is a peroxisomal biogenesis disorder caused by mutations in genes required for peroxisome formation (PEX genes).
• Peroxisomes are essential for peroxisomal oxidation, which involves the breakdown of:
o Very long-chain fatty acids (VLCFAs) (≥22 carbons).
o Branched-chain fatty acids (e.g., phytanic acid) through alpha oxidation.
• In Zellweger syndrome, the peroxisomal function is impaired, leading to the accumulation of VLCFAs and branched-chain fatty acids, which causes severe neurological, hepatic, and renal dysfunction.
Incorrect Options:
B. Alpha oxidation:
o Alpha oxidation of branched-chain fatty acids (e.g., phytanic acid) occurs in peroxisomes.
o While alpha oxidation is disrupted in Zellweger syndrome, it is only one aspect of the broader defect in peroxisomal oxidation.
C. Beta oxidation:
o Mitochondrial beta-oxidation of medium- and short-chain fatty acids is unaffected in Zellweger syndrome.
o However, peroxisomal beta-oxidation of VLCFAs is impaired, making “peroxisomal oxidation” the broader and more accurate answer.
D. Omega oxidation:
o Omega oxidation is a minor pathway for fatty acid metabolism that occurs in the endoplasmic reticulum, not the peroxisomes. It is unaffected in Zellweger syndrome.
E. Ketogenesis:
o Ketogenesis occurs in the liver mitochondria and is unrelated to peroxisomal function. It is not affected in Zellweger syndrome.
Key Takeaway:
Zellweger syndrome primarily affects peroxisomal oxidation, leading to the accumulation of very long-chain fatty acids and branched-chain fatty acids, which contributes to the clinical manifestations of the disorder.
24. A 30-year-old woman presents to the emergency department with complaints of nausea, vomiting, and abdominal pain. She has a history of type 1 diabetes and has not been taking her insulin regularly. Her breath has a fruity odor.
Which of the following is the most likely cause of her symptoms?
A. Hypoglycemia
B. Diabetic ketoacidosis
C. Lactic acidosis
D. Hyperglycemia
E. Respiratory alkalosis
Correct Answer: B. Diabetic ketoacidosis
Explanation:
• Diabetic ketoacidosis (DKA) is a life-threatening complication of uncontrolled type 1 diabetes.
• The fruity odor of her breath is caused by the accumulation of acetone, a byproduct of ketogenesis.
• In DKA, the absence of insulin leads to:
1. Uncontrolled lipolysis, resulting in an excess of free fatty acids.
2. Conversion of fatty acids to ketone bodies (acetoacetate, beta-hydroxybutyrate, and acetone) in the liver.
3. Ketoacidosis, as ketone bodies are acidic and lower blood pH.
• Symptoms such as nausea, vomiting, and abdominal pain are common in DKA, along with hyperglycemia due to a lack of insulin-mediated glucose uptake.
Incorrect Options:
A. Hypoglycemia:
o Hypoglycemia can occur in patients with diabetes but is associated with symptoms like confusion, sweating, and tremors, not fruity breath or abdominal pain.
o In this case, the patient has not been taking her insulin, making hyperglycemia more likely.
C. Lactic acidosis:
o Lactic acidosis can occur in conditions like sepsis, hypoxia, or metformin use in type 2 diabetes.
o While acidosis is present in DKA, it is due to ketones, not lactic acid.
D. Hyperglycemia:
o While hyperglycemia is a feature of DKA, it is not the primary cause of her symptoms. The fruity odor and acidosis point specifically to ketoacidosis, not just high blood sugar.
E. Respiratory alkalosis:
o Respiratory alkalosis occurs in conditions like anxiety or hyperventilation.
o In DKA, patients may have Kussmaul breathing, which is a compensatory response to metabolic acidosis, not alkalosis.
Key Takeaway:
The combination of fruity breath, abdominal pain, and nausea in a patient with a history of type 1 diabetes and missed insulin doses is characteristic of diabetic ketoacidosis (DKA), caused by the accumulation of ketone bodies due to insulin deficiency.
25. A 45-year-old man with uncontrolled diabetes presents with fruity-smelling breath, lethargy, and deep, rapid breathing (Kussmaul respirations). Which of the following is the primary substrate for the production of ketone bodies in the liver?
A. Glucose
B. Acetyl-CoA
C. Glycerol
D. Pyruvate
E. Oxaloacetate
Correct Answer: B. Acetyl-CoA
Explanation:
• Acetyl-CoA is the primary substrate for ketogenesis in the liver. It is derived from β-oxidation of fatty acids during fasting or insulin deficiency.
• Excess acetyl-CoA, due to reduced TCA cycle activity (from depleted oxaloacetate used in gluconeogenesis), is converted to ketone bodies (acetoacetate, beta-hydroxybutyrate, and acetone).
• The ketone bodies are then released into the bloodstream and used as an alternative energy source by peripheral tissues like the brain and muscles.
Incorrect Options:
A. Glucose: Glucose is not used in ketogenesis. Instead, its depletion promotes ketogenesis as an alternative energy source.
C. Glycerol: Glycerol from lipolysis is used in gluconeogenesis, not ketogenesis.
D. Pyruvate: Pyruvate is a precursor for gluconeogenesis and the TCA cycle, not ketone body production.
E. Oxaloacetate: Oxaloacetate is required for the TCA cycle and gluconeogenesis but is typically depleted during conditions favoring ketogenesis.
26. Which of the following factors is most likely to increase the rate of ketogenesis in the liver?
A. High insulin levels
B. Increased malonyl-CoA concentration
C. Increased activity of carnitine palmitoyltransferase I (CPT1)
D. Increased cytoplasmic citrate concentration
E. Decreased β-oxidation of fatty acids
Correct Answer: C. Increased activity of carnitine palmitoyltransferase I (CPT1)
Explanation:
• CPT1 facilitates the transport of long-chain fatty acids into the mitochondria for β-oxidation, producing acetyl-CoA, the substrate for ketogenesis.
• Increased CPT1 activity occurs when insulin levels are low, and malonyl-CoA levels decrease, favoring fatty acid oxidation and ketone body production.
Incorrect Options:
A. High insulin levels: Insulin inhibits ketogenesis by promoting lipogenesis and suppressing β-oxidation.
B. Increased malonyl-CoA concentration: Malonyl-CoA inhibits CPT1, reducing fatty acid oxidation and ketogenesis.
D. Increased cytoplasmic citrate concentration: Elevated citrate levels promote fatty acid synthesis, not ketogenesis.
E. Decreased β-oxidation of fatty acids: β-oxidation is essential for producing acetyl-CoA for ketogenesis. Decreased β-oxidation would suppress ketone body formation.
Key Takeaway:
• Ketogenesis is driven by low insulin levels, enhanced β-oxidation of fatty acids, and the transport of fatty acids into mitochondria by CPT1.
• Acetyl-CoA accumulation from fatty acid oxidation serves as the primary substrate for ketone body production.
27. In omega (ω) oxidation, which organelle is involved, and what is the primary product?
A. Mitochondria; Acetyl-CoA
B. Endoplasmic reticulum; Dicarboxylic acids
C. Peroxisomes; Ketone bodies
D. Cytoplasm; Malonyl-CoA
E. Nucleus; Fatty acid esters
Correct Answer: B. Endoplasmic reticulum; Dicarboxylic acids
Explanation:
• Omega (ω) oxidation occurs in the endoplasmic reticulum, targeting the terminal omega carbon of fatty acids.
• The primary products are dicarboxylic acids, which may be further processed or excreted in the urine, especially during mitochondrial β-oxidation defects. All other options are incorrect.
28. Which of the following conditions most likely increases omega (ω) oxidation activity?
A. Normal fasting state
B. Excess insulin
C. Mitochondrial β-oxidation defects
D. Ketogenic diet
E. Glucose-rich diet
Correct Answer: C. Mitochondrial β-oxidation defects
Explanation:
• When mitochondrial β-oxidation is impaired (e.g., in carnitine deficiency or medium-chain acyl-CoA dehydrogenase deficiency), omega oxidation becomes more active as a compensatory pathway to partially metabolize fatty acids.
• This results in the accumulation of dicarboxylic acids, a hallmark of increased omega oxidation.
All other options are incorrect.
29. What is the fate of dicarboxylic acids produced in omega (ω) oxidation?
A. Converted to glucose
B. Excreted in the urine or undergoes β-oxidation
C. Used for ketogenesis
D. Stored as triglycerides
E. Directly enters the TCA cycle
Correct Answer: B. Excreted in the urine or undergoes β-oxidation
Explanation:
• Dicarboxylic acids produced in omega oxidation can be:
1. Excreted in the urine (a diagnostic marker of omega oxidation).
2. Further oxidized via mitochondrial β-oxidation if the pathway is functional.
All other options are incorrect.
Key Takeaway:
Omega (ω) oxidation occurs in the endoplasmic reticulum, producing dicarboxylic acids as a backup pathway during mitochondrial β-oxidation defects or high levels of unmetabolized fatty acids.
30. Which of the following is the immediate precursor for triglyceride (TG) synthesis in adipose tissue?
A. Glycerol-3-phosphate and fatty acyl-CoA
B. Malonyl-CoA and fatty acyl-CoA
C. Acetyl-CoA and glycerol
D. Citrate and fatty acids
E. Pyruvate and acetyl-CoA
Correct Answer: A. Glycerol-3-phosphate and fatty acyl-CoA
Explanation:
• Triglyceride (TG) synthesis begins with glycerol-3-phosphate, which combines with three molecules of fatty acyl-CoA in a stepwise fashion to form triglycerides.
• Adipose tissue uses glycerol-3-phosphate derived from glycolysis, as it lacks glycerol kinase to directly utilize free glycerol.
All other options are incorrect.
31. Which hormone predominantly promotes triglyceride synthesis in adipose tissue?
A. Insulin
B. Glucagon
C. Cortisol
D. Epinephrine
E. Growth Hormone
Correct Answer: A. Insulin
Explanation:
• Insulin promotes triglyceride synthesis by:
1. Stimulating glucose uptake into adipose tissue via GLUT4, increasing glycerol-3-phosphate availability.
2. Activating lipoprotein lipase (LPL), which hydrolyzes circulating triglycerides into free fatty acids for uptake and storage as TG.
o Other hormones
All other options are incorrect.
32. Where does the majority of triglyceride synthesis occur during the well-fed state?
A. Adipose tissue
B. Liver
C. Skeletal muscle
D. Pancreas
E. Kidney
Correct Answer: B. Liver
Explanation:
• The liver is the primary site of triglyceride synthesis during the well-fed state.
• TGs synthesized in the liver are packaged into VLDL and transported to peripheral tissues, including adipose tissue, for storage.
• Adipose tissue also synthesizes TGs but relies on fatty acids and glycerol-3-phosphate delivered from the liver and other tissues.
Key Takeaway:
Triglyceride synthesis is a process requiring glycerol-3-phosphate and fatty acyl-CoA, promoted by insulin, and primarily occurs in the liver during the well-fed state, with significant storage in adipose tissue.
Author:Namrata Chhabra
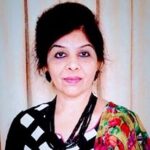