Library
Case-Based Multiple-Choice Questions on DNA Structure and Organization
- March 4, 2025
- Posted by: Namrata Chhabra
- Category: Library Learning resources Molecular Biology Molecular Biology Multiple-Choice questions Multiple-choice questions Practice questions Question Bank Question Bank Quizzes USMLE Content USMLE Style questions USMLE styled question bank
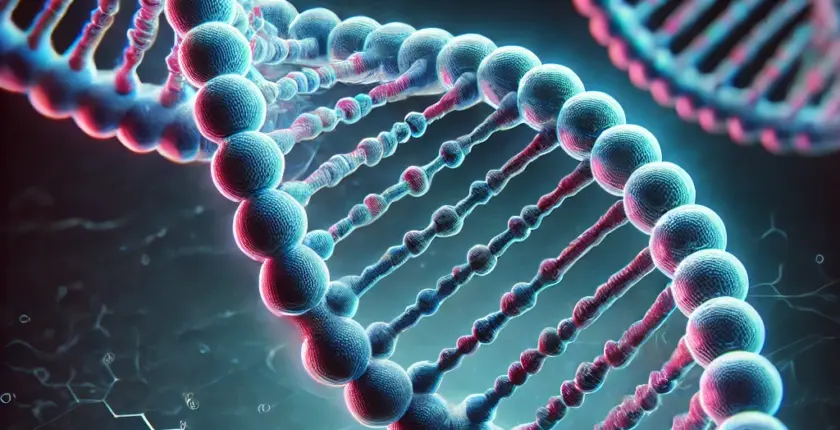
Case 1: DNA Stability and Mutations
A scientist is studying the stability of DNA in extreme environments. She observes that DNA isolated from a thermophilic bacterium has a higher melting temperature than DNA from a mesophilic organism. Upon further analysis, she notes a significantly different base composition between the two species.
Question:
What is the most likely explanation for the higher melting temperature of the thermophilic bacterium’s DNA?
A. It contains more histones, stabilizing the double helix.
B. It has a different sugar in its nucleotides, increasing stability.
C. It has a higher GC content, leading to more hydrogen bonds.
D. It has a longer DNA sequence, making it harder to denature.
E. It has more mutations, leading to stronger base-pair interactions.
Explanation:
A. It contains more histones, stabilizing the double helix.
• Incorrect. Histones play a role in DNA packaging in eukaryotic cells, but bacteria do not have histones in the same way. DNA stability in prokaryotes is primarily influenced by base composition.
B. It has a different sugar in its nucleotides, increasing stability.
• Incorrect. Both thermophilic and mesophilic bacteria use deoxyribose in their DNA. The sugar remains the same across different organisms.
C. It has a higher GC content, leading to more hydrogen bonds.
• Correct. Guanine (G) and Cytosine (C) pairs form three hydrogen bonds, compared to the two hydrogen bonds in Adenine (A)-Thymine (T) pairs. A higher GC content increases DNA stability and requires more heat to denature.
D. It has a longer DNA sequence, making it harder to denature.
• Incorrect. The length of DNA does not significantly impact melting temperature; rather, the composition of base pairs determines stability.
E. It has more mutations, leading to stronger base-pair interactions.
• Incorrect. Mutations do not necessarily strengthen DNA stability; instead, they introduce changes that may disrupt base-pairing rather than stabilize it.
Case 2: RNA Stability and Function
A researcher is investigating a virus that uses RNA instead of DNA for its genetic material. She finds that this RNA genome is extremely unstable at high temperatures, requiring special proteins to maintain its integrity. Additionally, the virus synthesizes a complementary RNA strand for replication.
Question:
Which structural feature makes RNA less stable than DNA, contributing to its instability?
A. The absence of hydrogen bonding between complementary bases.
B. The presence of ribose sugar instead of deoxyribose.
C. The ability to form a double-stranded helix similar to DNA.
D. The presence of disulfide bonds between bases.
E. The presence of uracil instead of thymine.
Explanation:
A. The absence of hydrogen bonding between complementary bases.
• Incorrect. RNA does exhibit base-pairing, particularly in double-stranded RNA (dsRNA) and secondary structures like hairpins, but this does not prevent its inherent instability due to its sugar structure.
B. The presence of ribose sugar instead of deoxyribose.
• Correct. RNA contains ribose, which has a hydroxyl (-OH) group at the 2′ carbon, making it more susceptible to hydrolysis and degradation, especially in high temperatures.
C. The ability to form a double-stranded helix similar to DNA.
• Incorrect. Most RNA is single-stranded, which makes it more prone to degradation than the double-stranded structure of DNA. Even double-stranded RNA (dsRNA) is less stable than DNA.
D. The presence of disulfide bonds between bases.
• Incorrect. RNA does not form disulfide bonds; instead, it relies on hydrogen bonding for base-pairing interactions.
E. The presence of uracil instead of thymine.
• Incorrect. While uracil replaces thymine in RNA, this change does not significantly affect stability. The instability is due to the sugar backbone, not the nitrogenous bases.
Case 3: Base Pairing and DNA Mutations
A geneticist is studying mutations in a DNA sequence associated with a rare genetic disorder. She finds that a point mutation has replaced an adenine (A) with a guanine (G) in one strand of the DNA. She analyzes how this mutation affects base pairing and the overall stability of the DNA helix.
Question:
How does replacing an adenine (A) with a guanine (G) affect base pairing in DNA?
A. It disrupts the hydrogen bonding because G cannot pair with T.
B. It increases the number of hydrogen bonds, stabilizing the DNA helix.
C. It allows Watson-Crick base pairing to continue without any changes.
D. It prevents the complementary strand from binding, leading to single-stranded DNA.
E. It weakens the DNA structure because G-T pairs form stronger bonds than A-T.
Explanation:
A. It disrupts the hydrogen bonding because G cannot pair with T.
• Correct. In normal Watson-Crick base pairing, adenine (A) pairs with thymine (T), and guanine (G) pairs with cytosine (C). A substitution of A with G means the new guanine (G) would be incorrectly paired with thymine (T), which does not form stable hydrogen bonds, leading to base-pairing disruption.
B. It increases the number of hydrogen bonds, stabilizing the DNA helix.
• Incorrect. While G-C pairs form three hydrogen bonds and are more stable than A-T pairs, in this case, the mutation leads to a mismatched G-T pair, which does not increase stability.
C. It allows Watson-Crick base pairing to continue without any changes.
• Incorrect. Watson-Crick base pairing is highly specific (A-T and G-C). A substitution from A to G disrupts this rule, causing a mismatch.
D. It prevents the complementary strand from binding, leading to single-stranded DNA.
• Incorrect. The complementary strand will still bind, but the mismatch may lead to errors during DNA replication or repair.
E. It weakens the DNA structure because G-T pairs form stronger bonds than A-T.
• Incorrect. G-T base pairs do not form stronger bonds; rather, they form weaker, unstable interactions that can lead to replication errors.
Case 4: RNA Base Pairing and Secondary Structures
A molecular biologist is studying an RNA virus that forms stable secondary structures. She observes that the virus’s genome contains regions rich in guanine (G) and cytosine (C) and notices that these regions contribute to the stability of the RNA molecule.
Question:
Why do GC-rich regions contribute to RNA stability?
A. GC pairs cause RNA to degrade faster, allowing for rapid turnover.
B. GC pairs do not participate in base pairing, leaving RNA single-stranded.
C. GC pairs form three hydrogen bonds, making them more stable than AU pairs.
D. GC pairs prevent RNA from folding into secondary structures.
E. GC pairs repel each other, preventing RNA from breaking apart.
Explanation:
A. GC pairs cause RNA to degrade faster, allowing for rapid turnover.
• Incorrect. GC-rich regions actually slow down degradation because the strong hydrogen bonds make it harder for nucleases to break the RNA apart.
B. GC pairs do not participate in base pairing, leaving RNA single-stranded.
• Incorrect. GC pairs are crucial for RNA secondary structures, such as stem-loops and hairpins, which contribute to RNA stability.
C. GC pairs form three hydrogen bonds, making them more stable than AU pairs.
• Correct. In RNA, guanine (G) pairs with cytosine (C), forming three hydrogen bonds, while adenine (A) pairs with uracil (U), forming only two. The additional hydrogen bond in GC pairs increases the stability of RNA, especially in high-temperature environments.
D. GC pairs prevent RNA from folding into secondary structures.
• Incorrect. GC pairs are essential in forming stable secondary structures, such as hairpin loops and double-stranded RNA regions, which are common in functional RNA molecules.
E. GC pairs repel each other, preventing RNA from breaking apart.
• Incorrect. GC base pairs attract each other through hydrogen bonding, not repulsion. This attraction helps stabilize RNA secondary structures.
Case 5: Major and Minor Grooves in DNA Binding
A molecular biologist is studying how transcription factors recognize specific DNA sequences. She observes that certain proteins bind more strongly to the major groove of DNA rather than the minor groove.
Question:
Why do most DNA-binding proteins interact with the major groove rather than the minor groove?
A. The major groove exposes more functional groups, allowing for specific interactions.
B. The major groove has a higher number of phosphodiester bonds, strengthening binding.
C. The major groove is wider, making it easier for proteins to fit inside.
D. The minor groove is too unstable to allow protein binding.
E. The minor groove lacks any hydrogen bonding sites, preventing interactions.
Explanation:
A. The major groove exposes more functional groups, allowing for specific interactions.
• Correct. The major groove provides greater access to the edges of base pairs, exposing functional groups that allow proteins to recognize specific DNA sequences through hydrogen bonding and van der Waals interactions.
B. The major groove has a higher number of phosphodiester bonds, strengthening binding.
• Incorrect. Phosphodiester bonds form the sugar-phosphate backbone and do not differ between grooves. They do not determine the binding preference of DNA-binding proteins.
C. The major groove is wider, making it easier for proteins to fit inside.
• Partially correct but not the main reason. While the major groove is indeed wider, the key factor is the availability of functional groups for sequence-specific recognition.
D. The minor groove is too unstable to allow protein binding.
• Incorrect. The minor groove is not inherently unstable. Some proteins, like certain antibiotics and histones, do bind to the minor groove, but it generally provides less sequence-specific information than the major groove.
E. The minor groove lacks any hydrogen bonding sites, preventing interactions.
• Incorrect. The minor groove does have hydrogen bonding sites, but they are fewer and less accessible compared to the major groove, making sequence recognition more difficult.
Case 6: Role of DNA Grooves in Drug Binding
A pharmaceutical company is developing a new drug that selectively binds to the minor groove of DNA to inhibit bacterial transcription. The researchers find that the drug is effective in binding without disrupting the DNA structure.
Question:
Why might a drug be designed to bind to the minor groove instead of the major groove?
A. The minor groove contains more sequence-specific recognition sites than the major groove.
B. The minor groove has a higher concentration of negatively charged phosphates.
C. The minor groove is narrower, allowing small molecules to fit without distorting DNA.
D. The minor groove is the only site where transcription factors bind.
E. The minor groove prevents hydrogen bonding with small molecules, increasing drug stability.
Explanation:
A. The minor groove contains more sequence-specific recognition sites than the major groove.
• Incorrect. The major groove provides more sequence-specific recognition sites, while the minor groove is typically recognized by small molecules through shape complementarity and electrostatic interactions rather than specific base-pair sequences.
B. The minor groove has a higher concentration of negatively charged phosphates.
• Incorrect. The phosphate groups are located on the DNA backbone, not within the grooves, and their distribution does not favor minor groove binding over major groove binding.
C. The minor groove is narrower, allowing small molecules to fit without distorting DNA.
• Correct. Many small-molecule drugs, such as distamycin and netropsin, bind to the minor groove because it provides a tight fit for small molecules without significantly altering the DNA structure.
D. The minor groove is the only site where transcription factors bind.
• Incorrect. Most transcription factors bind to the major groove, as it provides more chemical information for sequence-specific interactions. Some proteins, like TBP (TATA-binding protein), do bind to the minor groove, but this is an exception rather than the rule.
E. The minor groove prevents hydrogen bonding with small molecules, increasing drug stability.
• Incorrect. Small molecules can form hydrogen bonds with atoms in the minor groove, contributing to their binding specificity and affinity.
Case 7: Clinical Significance of Adenosine in Cardiovascular Function
A 55-year-old patient is admitted to the emergency department with complaints of palpitations and dizziness. An ECG reveals paroxysmal supraventricular tachycardia (PSVT). The attending physician administers an intravenous bolus of adenosine, which successfully restores normal sinus rhythm.
Question:
Why is adenosine used as a treatment for supraventricular tachycardia (SVT)?
A. Adenosine decreases conduction through the atrioventricular (AV) node, terminating reentrant circuits.
B. Adenosine directly inhibits the sinoatrial (SA) node, preventing further atrial contractions.
C. Adenosine increases calcium influx in cardiac muscle, strengthening contractions.
D. Adenosine stimulates adrenergic receptors, increasing heart rate to counteract SVT.
E. Adenosine permanently blocks electrical conduction, preventing future tachycardia episodes.
Explanation:
A. Adenosine decreases conduction through the atrioventricular (AV) node, terminating reentrant circuits.
• Correct. Adenosine acts on A1 receptors in the AV node, temporarily hyperpolarizing the tissue and slowing conduction. This interrupts reentrant circuits, effectively terminating SVT.
B. Adenosine directly inhibits the sinoatrial (SA) node, preventing further atrial contractions.
• Incorrect. Adenosine mainly affects the AV node rather than the SA node. The SA node may slow transiently, but the primary effect is on AV nodal conduction.
C. Adenosine increases calcium influx in cardiac muscle, strengthening contractions.
• Incorrect. Adenosine actually decreases calcium influx by inhibiting cyclic AMP-dependent pathways, leading to a temporary decrease in cardiac excitability.
D. Adenosine stimulates adrenergic receptors, increasing heart rate to counteract SVT.
• Incorrect. Adenosine does not stimulate adrenergic receptors; instead, it has an inhibitory effect on cardiac conduction.
E. Adenosine permanently blocks electrical conduction, preventing future tachycardia episodes.
• Incorrect. Adenosine has a very short half-life (less than 10 seconds) and only provides temporary AV nodal blockade. It does not prevent future episodes of SVT.
Case 8: Biological Significance of Adenosine in Energy Metabolism
A biochemist is studying energy metabolism in muscle cells. She notices that during periods of prolonged exercise, adenosine levels increase in the extracellular space. She hypothesizes that this increase plays a role in regulating cellular energy demands and vascular function.
Question:
What is the biological significance of adenosine accumulation during prolonged exercise?
A. Adenosine acts as a vasodilator, increasing blood flow to meet oxygen demands.
B. Adenosine binds to mitochondrial ATP synthase, accelerating ATP production.
C. Adenosine enhances glycolysis by directly phosphorylating glucose molecules.
D. Adenosine inhibits glycogen breakdown, conserving energy stores for later use.
E. Adenosine stimulates norepinephrine release, promoting muscle contraction.
Explanation:
A. Adenosine acts as a vasodilator, increasing blood flow to meet oxygen demands.
• Correct. Adenosine binds to A2A receptors on vascular smooth muscle, leading to vasodilation. This increases blood supply to active muscles, ensuring adequate oxygen and nutrient delivery.
B. Adenosine binds to mitochondrial ATP synthase, accelerating ATP production.
• Incorrect. ATP production is regulated by the electron transport chain, and adenosine does not directly bind to ATP synthase to enhance energy production.
C. Adenosine enhances glycolysis by directly phosphorylating glucose molecules.
• Incorrect. Adenosine itself does not phosphorylate glucose; rather, ATP (which contains adenosine) is the actual phosphorylating agent in glycolysis.
D. Adenosine inhibits glycogen breakdown, conserving energy stores for later use.
• Incorrect. Adenosine does not inhibit glycogenolysis; instead, it plays a role in energy regulation and vasodilation during metabolic stress.
E. Adenosine stimulates norepinephrine release, promoting muscle contraction.
• Incorrect. Adenosine generally has an inhibitory effect on neurotransmitter release, including norepinephrine, rather than stimulating it.
Case 9: Effect of AZT on DNA Synthesis
A first-year medical student is learning about the structure of DNA and how certain drugs can interfere with its synthesis. The instructor explains that zidovudine (AZT) is a drug used to treat HIV because it prevents the virus from making DNA from its RNA.
Question:
Why does AZT stop DNA synthesis in HIV?
A. AZT causes the DNA strands to break apart.
B. AZT changes the shape of DNA, making it unreadable.
C. AZT inhibits the formation of 3′-5′ phosphodiester bonds, stopping DNA elongation.
D. AZT removes nucleotides from the growing DNA strand.
E. AZT strengthens the DNA backbone, making it harder for the virus to replicate.
Explanation:
A. AZT causes the DNA strands to break apart.
• Incorrect. AZT does not break DNA strands; rather, it stops them from growing by blocking the addition of new nucleotides.
B. AZT changes the shape of DNA, making it unreadable.
• Incorrect. AZT does not alter the overall structure of DNA; it only prevents elongation during replication.
C. AZT inhibits the formation of 3′-5′ phosphodiester bonds, stopping DNA elongation.
• Correct. AZT mimics thymidine but lacks the hydroxyl (-OH) group at the 3′ position. Without this -OH group, new nucleotides cannot attach, preventing the formation of 3′-5′ phosphodiester bonds and stopping DNA synthesis.
D. AZT removes nucleotides from the growing DNA strand.
• Incorrect. AZT does not remove nucleotides; instead, it prevents new ones from being added.
E. AZT strengthens the DNA backbone, making it harder for the virus to replicate.
• Incorrect. AZT does not strengthen DNA; it blocks DNA synthesis by preventing elongation.
Case 10: Effect of DDI on DNA Replication
A researcher is studying the effects of didanosine (DDI), another drug used to treat HIV. Like AZT, DDI works by stopping viral replication. However, the researcher notices that DDI is an analog of deoxyadenosine instead of thymidine.
Question:
How does DDI prevent the formation of new viral DNA?
A. DDI blocks 3′-5′ phosphodiester bond formation, stopping DNA synthesis.
B. DDI removes adenine bases from the DNA, making it unstable.
C. DDI breaks hydrogen bonds between base pairs, causing DNA to fall apart.
D. DDI stops transcription by binding to viral RNA polymerase.
E. DDI increases the number of mutations in the viral DNA, making it nonfunctional.
Explanation:
A. DDI blocks 3′-5′ phosphodiester bond formation, stopping DNA synthesis.
• Correct. DDI is a nucleoside analog of deoxyadenosine but lacks a 3′-OH group. This prevents the addition of the next nucleotide by DNA polymerase, stopping DNA replication.
B. DDI removes adenine bases from the DNA, making it unstable.
• Incorrect. DDI does not remove bases; instead, it prevents new nucleotides from being added to the growing DNA chain.
C. DDI breaks hydrogen bonds between base pairs, causing DNA to fall apart.
• Incorrect. Hydrogen bonds hold the two DNA strands together, but DDI does not interfere with them; it stops replication by blocking phosphodiester bond formation.
D. DDI stops transcription by binding to viral RNA polymerase.
• Incorrect. DDI affects DNA synthesis, not RNA synthesis. It targets reverse transcriptase, the enzyme responsible for converting viral RNA into DNA.
E. DDI increases the number of mutations in the viral DNA, making it nonfunctional.
• Incorrect. DDI does not cause mutations; it prevents DNA elongation by stopping 3′-5′ phosphodiester bond formation.
Case 11: Mechanism of Action of 5-Fluorouracil (5-FU) in Cancer Treatment
A patient diagnosed with colorectal cancer is prescribed 5-fluorouracil (5-FU) as part of chemotherapy. The oncologist explains that 5-FU is a nucleoside analog that disrupts DNA synthesis.
Question:
How does 5-fluorouracil (5-FU) interfere with cancer cell growth?
A. 5-FU increases the production of thymine, enhancing DNA replication.
B. 5-FU inhibits thymidylate synthase, reducing thymidine levels and blocking DNA synthesis.
C. 5-FU prevents DNA unwinding by interfering with helicase enzymes.
D. 5-FU removes uracil from RNA, preventing proper transcription.
E. 5-FU strengthens hydrogen bonding between base pairs, making DNA more stable.
Explanation:
A. 5-FU increases the production of thymine, enhancing DNA replication.
• Incorrect. 5-FU actually inhibits the production of thymidine, not increases it. Without thymidine, cells cannot properly synthesize DNA.
B. 5-FU inhibits thymidylate synthase, reducing thymidine levels and blocking DNA synthesis.
• Correct. 5-FU is a uracil analog that binds and inhibits thymidylate synthase, preventing the conversion of uracil to thymidine. Without thymidine, rapidly dividing cancer cells cannot replicate their DNA, leading to cell death.
C. 5-FU prevents DNA unwinding by interfering with helicase enzymes.
• Incorrect. 5-FU does not affect helicase enzymes. Its main action is blocking thymidine synthesis.
D. 5-FU removes uracil from RNA, preventing proper transcription.
• Incorrect. 5-FU does not remove uracil from RNA but rather disrupts DNA synthesis by mimicking uracil and inhibiting thymidylate synthase.
E. 5-FU strengthens hydrogen bonding between base pairs, making DNA more stable.
• Incorrect. 5-FU does not strengthen DNA stability; it disrupts nucleotide synthesis, preventing proper DNA replication.
Case 12: Cytarabine (Ara-C) and Its Role in Cancer Therapy
A leukemia patient is being treated with cytarabine (Ara-C), a drug that mimics cytidine. The oncologist explains that Ara-C is a nucleoside analog used to target rapidly dividing cancer cells.
Question:
How does cytarabine (Ara-C) inhibit cancer cell proliferation?
A. Ara-C adds extra cytosine bases to DNA, increasing replication speed.
B. Ara-C enhances ribosome function, increasing protein production in cancer cells.
C. Ara-C incorporates into DNA but prevents the formation of 3′-5′ phosphodiester bonds.
D. Ara-C stabilizes the DNA double helix, making it resistant to mutations.
E. Ara-C strengthens the nuclear membrane, preventing DNA from being accessed.
Explanation:
A. Ara-C adds extra cytosine bases to DNA, increasing replication speed.
• Incorrect. Ara-C does not increase replication but rather inhibits it by preventing proper nucleotide linkage.
B. Ara-C enhances ribosome function, increasing protein production in cancer cells.
• Incorrect. Ara-C does not affect ribosomes or protein synthesis directly; it targets DNA replication.
C. Ara-C incorporates into DNA but prevents the formation of 3′-5′ phosphodiester bonds.
• Correct. Ara-C is a cytidine analog that gets incorporated into the DNA strand. However, it lacks the correct structure for forming 3′-5′ phosphodiester bonds, preventing DNA elongation and leading to cell death.
D. Ara-C stabilizes the DNA double helix, making it resistant to mutations.
• Incorrect. Ara-C does not stabilize DNA; it disrupts DNA synthesis by blocking chain elongation.
E. Ara-C strengthens the nuclear membrane, preventing DNA from being accessed.
• Incorrect. Ara-C does not affect the nuclear membrane; it interferes with DNA replication inside the nucleus.
Case 13: Role of Histone Acetylation in Gene Expression
A researcher is studying how histone modifications affect gene expression. She treats a group of cells with a histone acetyltransferase (HAT) inhibitor and observes a decrease in the transcription of several genes.
Question:
How does histone acetylation influence gene expression?
A. Histone acetylation condenses chromatin, making genes less accessible for transcription.
B. Histone acetylation decreases the negative charge on histones, weakening their interaction with DNA.
C. Histone acetylation increases DNA methylation, leading to gene silencing.
D. Histone acetylation prevents RNA polymerase from binding to the promoter region.
E. Histone acetylation removes histones from DNA, exposing the entire genome for transcription.
Explanation:
A. Histone acetylation condenses chromatin, making genes less accessible for transcription.
• Incorrect. Acetylation loosens chromatin structure, increasing gene accessibility rather than condensing it.
B. Histone acetylation decreases the negative charge on histones, weakening their interaction with DNA.
• Correct. Histones are positively charged due to lysine residues, which strongly bind to negatively charged DNA. Acetylation neutralizes these positive charges, weakening DNA-histone interactions and making DNA more accessible for transcription.
C. Histone acetylation increases DNA methylation, leading to gene silencing.
• Incorrect. Acetylation generally promotes gene activation, whereas DNA methylation is associated with gene silencing.
D. Histone acetylation prevents RNA polymerase from binding to the promoter region.
• Incorrect. Acetylation facilitates transcription by opening chromatin and allowing transcription factors and RNA polymerase to access the promoter region.
E. Histone acetylation removes histones from DNA, exposing the entire genome for transcription.
• Incorrect. Histones remain bound to DNA; acetylation simply loosens their grip, allowing specific genes to be transcribed.
Case 14: Role of Histone Methylation in Gene Regulation
A scientist is investigating the effects of histone methylation on gene expression. She finds that methylation of histone H3 at lysine 9 (H3K9) leads to reduced expression of nearby genes.
Question:
How does histone methylation affect gene regulation?
A. Histone methylation always activates gene expression by loosening chromatin.
B. Histone methylation creates a rigid chromatin structure that permanently silences genes.
C. Histone methylation can either activate or repress genes depending on the site of modification.
D. Histone methylation removes histones from DNA, making genes permanently active.
E. Histone methylation weakens DNA-histone interactions, allowing continuous transcription.
Explanation:
A. Histone methylation always activates gene expression by loosening chromatin.
• Incorrect. Histone methylation can either activate or repress gene expression, depending on which lysine or arginine residue is modified.
B. Histone methylation creates a rigid chromatin structure that permanently silences genes.
• Incorrect. While some methylation marks, such as H3K9me3, are associated with gene repression, they do not necessarily make chromatin permanently inactive.
C. Histone methylation can either activate or repress genes depending on the site of modification.
• Correct. Methylation of different histone residues has distinct effects. For example, H3K4 methylation is associated with gene activation, while H3K9 and H3K27 methylation are linked to gene repression.
D. Histone methylation removes histones from DNA, making genes permanently active.
• Incorrect. Methylation modifies histone proteins but does not remove them from DNA.
E. Histone methylation weakens DNA-histone interactions, allowing continuous transcription.
• Incorrect. Unlike acetylation, methylation does not always weaken DNA-histone interactions. Some methylation marks promote gene activation, while others reinforce chromatin compaction and gene silencing.
Case 15: Understanding Chargaff’s Rule in DNA Base Composition
A medical student is analyzing the nucleotide composition of a DNA sample. She finds that 30% of the bases are adenine (A). Using Chargaff’s rule, she calculates the percentages of the other bases.
Question:
According to Chargaff’s rule, what percentage of the DNA sample consists of cytosine (C)?
A. 20%
B. 30%
C. 40%
D. 50%
E. 60%
Explanation:
A. 20%
• Correct. According to Chargaff’s rule, the amount of adenine (A) is equal to thymine (T), and the amount of cytosine (C) is equal to guanine (G). Since the DNA contains 30% A, it must also contain 30% T. This leaves 40% of the bases for C and G, meaning each must be 20%.
B. 30%
• Incorrect. Cytosine (C) does not match adenine (A) in quantity; it pairs with guanine (G), and both together make up the remaining percentage.
C. 40%
• Incorrect. 40% represents the combined percentage of cytosine (C) and guanine (G), not cytosine alone.
D. 50%
• Incorrect. DNA is a double-stranded molecule where total purines (A + G) equal total pyrimidines (T + C), but no single base reaches 50%.
E. 60%
• Incorrect. The total of all four bases must equal 100%, and cytosine cannot be more than guanine.
Case 16: Application of Chargaff’s Rule to Different Organisms
A researcher is studying the DNA composition of a bacterial species and finds that the percentage of adenine (A) is significantly different from the percentage of thymine (T). She is surprised by this result and consults a colleague.
Question:
Why might Chargaff’s rule not apply in this case?
A. The organism has a single-stranded DNA genome.
B. The organism uses uracil instead of thymine in its DNA.
C. The organism lacks cytosine, altering its base composition.
D. The organism contains only purines (A and G) in its DNA.
E. The organism follows a non-standard genetic code, making Chargaff’s rule irrelevant.
Explanation:
A. The organism has a single-stranded DNA genome.
• Correct. Chargaff’s rule applies only to double-stranded DNA, where base pairing ensures equal amounts of A and T, and C and G. In single-stranded DNA (ssDNA) viruses or some bacteria with ssDNA genomes, there is no complementary base pairing, so the nucleotide composition can vary.
B. The organism uses uracil instead of thymine in its DNA.
• Incorrect. Uracil (U) is found in RNA, not DNA. Even if an organism used uracil instead of thymine, Chargaff’s rule would still apply in double-stranded nucleic acids.
C. The organism lacks cytosine, altering its base composition.
• Incorrect. Cytosine is a fundamental base in DNA. No known organism lacks cytosine in its genome.
D. The organism contains only purines (A and G) in its DNA.
• Incorrect. DNA must have both purines (A, G) and pyrimidines (C, T) to form a stable double-helix structure.
E. The organism follows a non-standard genetic code, making Chargaff’s rule irrelevant.
• Incorrect. The genetic code determines how codons translate into amino acids but does not affect base pairing rules in DNA.
Case 17: Gene Expression and Chromatin Remodeling in Cancer
A researcher is studying a type of aggressive cancer that shows widespread loss of heterochromatin and an increase in euchromatin-associated modifications.
Question:
What is the most likely consequence of excessive euchromatin formation in these cancer cells?
A. Increased expression of oncogenes, promoting uncontrolled cell growth.
B. Increased heterochromatin formation, leading to gene silencing.
C. Increased methylation of histones, reducing gene expression.
D. Reduced acetylation of histones, leading to tighter chromatin packing.
E. Suppression of transcription factors, preventing gene activation.
Explanation:
A. Increased expression of oncogenes, promoting uncontrolled cell growth.
• Correct. Euchromatin is associated with active transcription. If oncogenes (genes that drive cancer growth) are in euchromatic regions, their overexpression can lead to uncontrolled cell proliferation and tumor progression.
B. Increased heterochromatin formation, leading to gene silencing.
• Incorrect. The case describes loss of heterochromatin, not an increase. This means genes that should be silenced may become active.
C. Increased methylation of histones, reducing gene expression.
• Incorrect. Methylation is typically associated with heterochromatin formation and gene repression, but in this case, heterochromatin is reduced, so gene activation is more likely.
D. Reduced acetylation of histones, leading to tighter chromatin packing.
• Incorrect. Histone acetylation loosens chromatin structure to promote gene expression. The scenario describes increased euchromatin, which is associated with higher histone acetylation, not reduction.
E. Suppression of transcription factors, preventing gene activation.
• Incorrect. In euchromatin-rich cells, transcription factors have easier access to DNA, leading to increased gene activation rather than suppression.
Case 18: Role of Heterochromatin in X-Chromosome Inactivation
A physician is studying a patient with Turner syndrome (45, X0), a condition where individuals have only one X chromosome instead of the usual two. In a normal female (XX), one X chromosome is randomly inactivated to form Barr bodies, a form of facultative heterochromatin. However, in Turner syndrome, the patient lacks a second X chromosome.
Question:
What is the consequence of having only one active X chromosome with no heterochromatin-based X inactivation?
A. A normal phenotype, since one X chromosome is sufficient for gene expression.
B. Complete loss of gene expression from the X chromosome.
C. Increased expression of X-linked genes, leading to developmental abnormalities.
D. Silencing of all X-linked genes, preventing cell division.
E. The formation of two Barr bodies, compensating for the missing X chromosome.
Explanation:
A. A normal phenotype, since one X chromosome is sufficient for gene expression.
• Incorrect. While one X chromosome is essential, females typically inactivate one X to balance gene dosage with males (XY). In Turner syndrome, the lack of a second X leads to gene dosage imbalances and developmental abnormalities.
B. Complete loss of gene expression from the X chromosome.
• Incorrect. One X chromosome remains fully active, so genes are still transcribed. The problem arises from the missing second X, which would normally be inactivated as a Barr body in a healthy XX female.
C. Increased expression of X-linked genes, leading to developmental abnormalities.
• Correct. In a normal XX female, X-inactivation balances gene dosage between males (XY) and females (XX). In Turner syndrome (X0), there is no second X chromosome to inactivate, leading to higher-than-normal expression of some X-linked genes, causing developmental issues such as short stature, heart defects, and ovarian failure.
D. Silencing of all X-linked genes, preventing cell division.
• Incorrect. If all X-linked genes were silenced, the cells would not survive. In Turner syndrome, the single X chromosome remains active and functions, though improperly regulated.
E. The formation of two Barr bodies, compensating for the missing X chromosome.
• Incorrect. Barr bodies are formed from inactivated X chromosomes. Since there is only one X chromosome in Turner syndrome, no Barr bodies form.
Case 19: DNA Packaging and Its Role in Cell Division
A researcher is studying how DNA is packaged inside human cells. She observes that during interphase, DNA is loosely packed, allowing transcription, but during mitosis, it becomes highly condensed to facilitate chromosome segregation.
Question:
Why does DNA need to be highly condensed during mitosis?
A. Condensed DNA prevents genetic recombination during cell division.
B. Condensed DNA protects the genetic material from enzymatic degradation.
C. Condensed DNA prevents transcription, allowing the cell to focus on dividing.
D. Condensed DNA reduces tangling and ensures accurate chromosome separation.
E. Condensed DNA stabilizes nuclear pores, allowing proper mitotic spindle attachment.
Explanation:
A. Condensed DNA prevents genetic recombination during cell division.
• Incorrect. Genetic recombination (crossing over) occurs during meiosis, not mitosis. DNA condensation in mitosis is for organization, not recombination prevention.
B. Condensed DNA protects the genetic material from enzymatic degradation.
• Incorrect. DNA is generally stable in the nucleus, and condensation is primarily for structural organization, not protection.
C. Condensed DNA prevents transcription, allowing the cell to focus on dividing.
• Partially correct, but not the main reason. While transcription is largely halted during mitosis, the primary reason for condensation is efficient chromosome segregation, not transcription inhibition.
D. Condensed DNA reduces tangling and ensures accurate chromosome separation.
• Correct. Highly condensed chromosomes are organized and compact, making it easier for the mitotic spindle to accurately separate them into daughter cells without entanglement or errors.
E. Condensed DNA stabilizes nuclear pores, allowing proper mitotic spindle attachment.
• Incorrect. Nuclear pores disassemble during mitosis, and DNA packaging does not directly affect spindle attachment. The spindle attaches to kinetochores, not nuclear pores.
Case 20: Levels of DNA Packaging and Chromatin Structure
A medical student is learning about DNA organization in eukaryotic cells. She reads that DNA is packaged around histones to form nucleosomes, which further compact into chromatin fibers and chromosomes. She is curious about how DNA compaction varies in different cellular states.
Question:
Which statement best describes the relationship between DNA packaging and gene expression?
A. Euchromatin has tightly packed nucleosomes, preventing gene transcription.
B. Histones are absent in highly condensed chromatin, allowing tighter DNA folding.
C. Nucleosomes help organize DNA into loops, but they do not affect gene accessibility.
D. Tightly packed chromatin (heterochromatin) is transcriptionally inactive.
E. Unwound DNA (euchromatin) is unstable and must be fully condensed to function.
Explanation:
A. Euchromatin has tightly packed nucleosomes, preventing gene transcription.
• Incorrect. Euchromatin is loosely packed, allowing access to transcription machinery and facilitating active gene expression.
B. Histones are absent in highly condensed chromatin, allowing tighter DNA folding.
• Incorrect. Histones are essential for DNA packaging at all levels. Without histones, DNA would not compact efficiently.
C. Nucleosomes help organize DNA into loops, but they do not affect gene accessibility.
• Incorrect. Nucleosomes directly influence gene accessibility. Loosely packed nucleosomes in euchromatin make genes more accessible, while tightly packed nucleosomes in heterochromatin block transcription.
D. Tightly packed chromatin (heterochromatin) is transcriptionally inactive.
• Correct. Heterochromatin is highly condensed, making genes inaccessible to transcription factors and RNA polymerase. This packaging plays a key role in gene silencing.
E. Unwound DNA (euchromatin) is unstable and must be fully condensed to function.
• Incorrect. Euchromatin is essential for transcription. Although it is less condensed than heterochromatin, it remains structurally stable within the nucleus.
Author:Namrata Chhabra
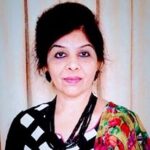